Phase Change Materials and Marangoni convection
Nearly every space mission must deal with significant fluctuations in temperature, caused, for example, by the heat generated by electrical and propulsion systems or the cyclic exposure to radiation fluxes. Small satellites, which are especially relevant nowadays, have particularly low thermal inertia and may suffer under extreme temperature changes. Designing efficient and effective thermal control systems is thus a critical demand in the space sector.
Organic PCMs are an attractive option for this purpose. However, some difficulties, including low heat transfer rates, stress damage resulting from volume changes, and the inconvenient formation of bubbles (voids) during melting, have discouraged wider use. In this context, most of the passive solutions that have been considered for improving heat transfer through the addition of conducting material bring with them the disadvantage of increased mass and/or size of the PCM device, and, by themselves, they do nothing to restore the convective motion that can significantly improve heat transfer. The thermocapillary effect, however, can act as an alternative heat transport mechanism to that of natural convection in microgravity [1].
The `Thermocapillary Effects in Phase change materials in Microgravity” (TEPiM) experiment recently confirmed this and motivated the development of the Effect of Marangoni convection on Heat Transfer in Phase Change Materials” (MarPCM) experiment. The main findings of TEPiM and current status of MarPCM are summarized here.
The "Thermocapillary Effects in Phase Change Materials in Microgravity" experiment
The TEPiM experiment investigated the melting dynamics and heat transfer characteristics of the alkane n-octadecane in the presence of thermocapillary convection in microgravity, with the principal objective of quantifying the contribution of thermocapillary flow to the heat transfer rate[2]. The experiment was conducted on parabolic flights, which provided repeated periods of microgravity lasting (approximately) 20 s, as part of the ESA educational program “Fly Your Thesis! 2016” during the 65th ESA Parabolic Flight campaign [3].
The core set-up of the experiment consisted of three pairs of cuboidal cells with variable height. Each pair included a Marangoni (thermocapillary) cell, which incorporated a layer of air above the PCM in order to support thermocapillary convection, and a reference cell containing only the PCM, wherein melting was dominated by thermal conduction (diffusion). Both types of cells were subjected to controlled thermal conditions, allowing for a direct comparison of the phase change in each scenario. The melting process was monitored with two cameras, six thermocouples and the airplane accelerometers.
Snapshots of TEPiM cells.
Prior to the microgravity period, the temperature along the lateral boundary was made approximately uniform at 80ºC. The subsequent (rapid) melting of the solid PCM produced a controlled liquid/air interface at the beginning of the microgravity period so that the development of thermocapillary convection could be concentrated during the available reduced gravity. As the solid PCM separating the liquid and the air layer melted, the liquid/air interface was created. The resulting thermocapillary convection increased the heat transfer rate and locally accelerated melting, as reflected in the rapid advance of the solid/liquid front (S/L) near the liquid/air interface [4].
Solid/liquid front evolution during a microgravity period: Marangoni vs. reference cell.
Overall, TEPiM demonstrated the potential of the thermocapillary effect to enhance heat transport in microgravity and suggested it could be a viable alternative for designing more efficient thermal control systems based on PCMs in future space missions.
The "Effect of Marangoni Convection on Heat Transfer in Phase Change Materials" experiment
The principal objective of the MarPCM project is to provide the necessary theoretical and experimental work needed to evaluate the effectiveness of thermocapillary convection and related strategies, like nanoparticles, for improving PCM devices and to support the development of the microgravity experiment planned for the ISS [5]. The general objectives are as follows:
- Quantify the effect of thermal Marangoni convection on the heat transfer rate (melting and solidification times) in comparison with the case of pure thermal diffusion (conduction).
- Compare the effectiveness of thermal Marangoni convection for a PCM with a cuboidal geometry (possessing one rectangular free surface) and for cylindrical geometry (a liquid bridge configuration when melted).
- Determine the dependence on temperature gradient of the contribution from thermal Marangoni convection, including the dependence on dynamical regimes.
- Evaluate the robustness of the proposed design and any practical difficulties associated with maintaining a free surface, and PCM performance in general, over a series of melting and freezing cycles.
- Compare the heat transport efficiency of a pure PCM with a NePCM (containing metallic nanoparticles.
- Investigate the effect of mechanical vibrations with and without thermal Marangoni convection.
In short, the experiment aims to definitively test heat enhancement strategies based on the thermocapillary effect during complete melting and solidification cycles, something that is only possible in the sustained microgravity environment of the ISS.
The scientific consortium encompasses three research groups: the ‘Fluid Mechanics’ group from Mondragon University, the ‘Digital Processing of Signals in Aerospace and Biomedics’ (SPABE) group from Universitat Rovira i Virgili, and the ‘Aerospace Science and Technology’ group from the Technical University of Madrid (UPM), which is coordinating the project.
To achieve these objectives, fixed temperatures will be applied to opposite ends of the PCM to establish a controlled melting or solidification process, which will be observed by means of optical cameras and appropriate illumination. The PCM samples will be held in containers constructed with two different geometries: cuboidal and cylindrical. The quantitative evaluation of the performance of different PCM cells will be made by comparing the evolution of the S/L front (equivalently, the liquid volume) over time; more effective heat transfer will manifest as shorter melting or solidification times. The set of recorded images will be complemented by thermal measurements at key positions along the cell, which will allow us to distinguish different dynamical regimes during melting and solidification processes.
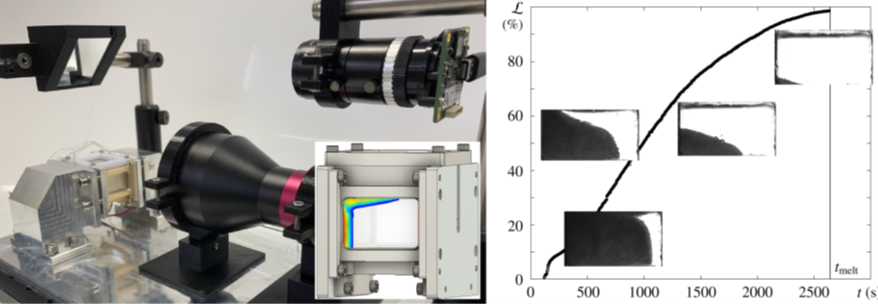
On left, MarPCM scientific prototype of the cuboidal Marangoni cell. On the right,Example of a ground experiment.
MarPCM is approved by ESA for execution on board the ISS. The experiment is expected to demonstrate that thermal Marangoni convection increases the heat transfer rate by a significant factor, depending on the physical properties of the PCM, its geometry, and the applied temperatures. The project is currently in the final design and manufacturing stages of the Engineering Model. Prototypes of the cuboidal and cylindrical geometries have been built for engineering and scientific testing on ground [6].
Selected references
- [1] P. Salgado Sánchez, J. M. Ezquerro, J. Fernández, and J. Rodríguez. Thermocapillary effects during the melting of phase change materials in microgravity: Heat transport enhancement. Int. J. Heat Mass Transf. 163, 120478 (2020).
- [2] J. M. Ezquerro, P. Salgado Sánchez, A. Bello, J. Rodríguez, V. Lapuerta, and A. Laverón-Simavilla. Experimental evidence of thermocapillarity in phase change materials in microgravity: Measuring the effect of Marangoni convection in solid/liquid phase transitions. Int. Comm. Heat Mass Transf. 113, 104529 (2020).
- [3] J. M. Ezquerro, A. Bello, P. Salgado Sánchez, A. Laverón-Simavilla, and V. Lapuerta. The Thermocapillary Effects in Phase Change Materials in Microgravity experiment: Design, preparation and execution of a parabolic flight experiment. Acta Astron. 162, 185-196 (2019).
- [4] P. Salgado Sánchez, J. M. Ezquerro, J. Porter, J. Fernández, and I. Tinao. Effect of thermocapillary convection on the melting of phase change materials in microgravity: Experiments and simulations. Int. J. Heat Mass Transf. 154, 119717 (2020).
- [5] J. Porter, A. Laverón-Simavilla, M. M. Bou-Ali, X. Ruiz, F. Gavalda, et al. The ‘‘Effect of Marangoni Convection on Heat Transfer in Phase Change Materials’’ experiment. Acta Astron. 210, 212-223 (2023).
- [6] U. Martínez, J. M. Ezquerro, J. Fernández, and K. Olfe. The “Effect of Marangoni Convection on Heat Transfer in Phase Change Materials” experiment: Design and performance of the cuboidal cell. Acta Astron. 216, 152-162 (2024)